Martian Halite and Preservation Potential of Biosignatures
By Crew Biologist Anushree Srivastava
First of all, what is halite?
Halite is natural salt – sodium chloride (NaCl) – or table salt. Halite is also commonly known as rock salt. Halite is formed or precipitated as a result of evaporation of salt lakes and seas, and in turn called evaporite. During evaporation, liquid water is transformed into gas and the halite precipitated. Halite makes cubic crystals and is found in sedimentary salt basins. Halite deposits are potential targets for astrobiological exploration of Mars due to its capability to capture organics and preserve them over a long period of time. Osterloo and co-workers detected more than 600 locations of chloride bearing deposits, including halite, on the surface of Martian Southern Hemisphere using the Thermal Emission Imaging System (THEMIS). These deposits date from Noachian (about 4.5 billion years ago, to about 3.7 billion years ago) to Hesperian (about 3.7 billion years ago to about 3 billion years ago): Martian geological timescale. The Noachian period is considered to be warmer and wetter and thus more conducive to life. Moreover, multiple geomorphological observations suggest the formation of these Martian evaporites through surface water runoff and evaporation and these deposits are considered to be contemporaneous to the age of the formation of valley networks on Mars. This is also suggestive to the last widespread water activity on the ancient Mars.
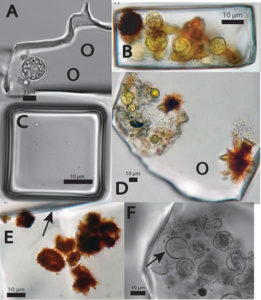
Halite and Fluid-inclusion.
Nice illustration by Lowenstein et al., 2011 “Photomicrographs of fluid inclusions in ancient halite from Saline Valley and Death Valley (Calif., USA) cores. (A) Dunaliella cell (left) and miniaturized prokaryotes (circled), in irregularly shaped fluid inclusion, Saline Valley core, 93 m, 150 ka. (B) Light green and orange Dunaliella cells suggest preservation of chlorophyll and β-carotene, Death Valley core, 17.8 m, 34 ka. Modified from Schubert et al. (2010). (C) Miniaturized prokaryotes in cubic fluid inclusion, Death Valley core 16.5 m, 31 ka. Modified from Schubert et al. (2009). (D) Portion of large fluid inclusion containing yellow-green Dunaliella cells and two cells coated with outward radiating crystals of β-carotene (brown). Miniaturized prokaryote is circled. Death Valley core 15.7 m, 29 ka. (E) Portion of fluid inclusion showing Dunaliella cells heavily coated with crystalline β-carotene, Death Valley core 15.7 m, 29 ka. Arrow shows the boundary between the fluid inclusion and the host halite crystal. (F) Dunaliella cells in various stages of degradation within a large fluid inclusion, Saline Valley core, 44 m, ca. 70 ka. Arrow shows ruptured glycocalyx (cell coat) of one Dunaliella cell.”
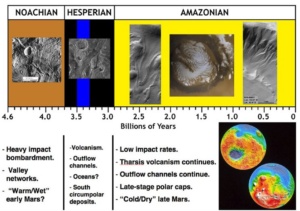
Martian Geological Timescale and corresponding features.
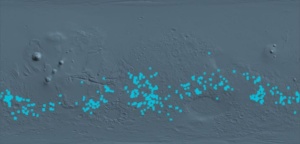
Global Mars Elevation Map by Osterloo et al (2010) indicating locations of chloride bearing deposits on the Southern Hemisphere of Mars.
Halite is capable of entraining and preserving carbon or biosignatures – any substance that is indicator of past or present life – through the mechanism called sequestration. Therefore, any microorganisms were surviving on the ancient Mars which was suitable for life could be captured inside halite. Furthermore, halite encases inclusions of the precipitating fluid which form a favourable micro-habitat to any microorganism trapped inside it during the growth phase of the halite crystals. Fluid-inclusions are rich of minerals and sometimes host algae which secrete glycerol. Therefore, any entrapped microorganism that can utilize these resources can survive a prolonged period of time (possible over thousands or million years) buried inside the halite crystals. Halite can also act as a shield for these microbes in order to protect them from high radiation. Studies have also found halite in multiple Martian meteorites such as Zag, and Monahans. Hence, halite should be one of the prime targets of the astrobiological exploration of Mars 2020 mission!
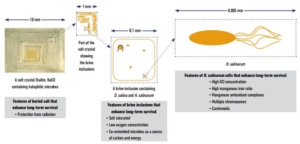
Schematic presentation of the features of halite and Halobacterium salinarum that facilitate long-term survival inside halite crystal (Credit: McGenity, 2014)
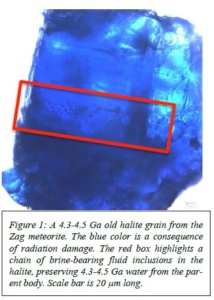
Zag: Martian meteorites hosting 4.3-4.5 Ga (billion years old) halite. (Credit: Fries et al, 2015)
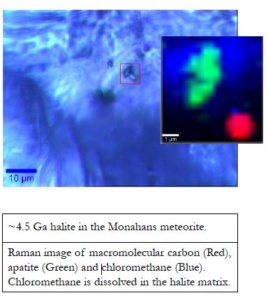
Monahans: Martian meteorites hosting ~4.5 Ga halite. (Credit: Zolensky 1999, Whitby, 2000, Bogard 2001)
Further reading:
DasSarma S (2006) Extreme Halophiles Are Models for Astrobiology. Microbe 1: 120-126
Davila AF et al. (2011) A large sedimentary basin in the Terra Sirenum region of the southern highlands. Icarus 212: 579–589
Davila AF, McKay CP (2011) Salt Flats in Terra Syrenum-A site to search for extant and extinct life on Mars. Analogue Sites for Mars Missions.
Fries et al (2015) Martian Halite: Potential for both Long-term Preservation of Organics and Source of Water. Abstract in “First Landing Site/Exploration Zone Workshop for Human Missions to the Surface of Mars”.
Gooding JL (1992) Soil mineralogy and chemistry on Mars: Possible clues from salts and clays in SNC meteorites. Icarus 99: 28-41
Gramain A, Chong Díaz GC, Demergasso C, Lowenstein TK, McGenity TJ (2011) Archaeal diversity along a subterranean salt core from the Salar Grande (Chile). Environmental Microbiology 13: 2105–2121
Landis GA (2001) Martian Water: Are there Extant Halobacteria on Mars? Astrobiology 1: 161-164
Lowenstein TK, Schubert BA, Timofeeff MN (2011) Microbial communities in fluid inclusions and long-term survival in halite. GSA Today 21: 4-9
McGenity T (2014) The immortal, halophilic superhero: Halobacterium salinarum – a long-lived poly-extremophile, Microbiology Today 24-27
Osterloo, MM et al. (2010). Geologic context of proposed chloride-bearing materials on Mars. Journal of Geophysical Research 115
Rieder R, Gellert R, Anderson RC, Brückner J, Clark BC, Dreibus G, Economou T, Klingelhöfer G, Lugmair GW, Ming DW, Squyres SW, d’Uston C, Wänke H, Yen A, Zipfel J (2004) Chemistry of Rocks and Soils at Meridiani Planum from the Alpha Particle X-ray Spectrometer. Science 306: 1746–1749
Schubert BA, Lowenstein TK, Timofeeff MN (2009) Microscopic identification of prokaryotes in modern and ancient halite, Saline Valley and Death Valley, California. Astrobiology 9: 467–482
Schubert BA, Lowenstein TK, Timofeeff MN, Parker MA (2010) Halophilic Archaea cultured from ancient halite, Death Valley, California. Environmental Microbiology 12: 440–454
Squyres SW, Knoll AH, Arvidson RE, et al. (2006) Two years at Meridiani Planum: results from the Opportunity Rover. Science 313: 1403–7
Treiman AH, Gleason JD, Bogard DD (2000) The SNC meteorites are from Mars. Planetary Space Sciences 48:1213–1230